Mechanisms that regulate localization of a DNA double-strand break to the nuclear periphery
- PMID: 19390086
- PMCID: PMC2675867
- DOI: 10.1101/gad.1782209
Mechanisms that regulate localization of a DNA double-strand break to the nuclear periphery
Abstract
DNA double-strand breaks (DSBs) are among the most deleterious forms of DNA lesions in cells. Here we induced site-specific DSBs in yeast cells and monitored chromatin dynamics surrounding the DSB using Chromosome Conformation Capture (3C). We find that formation of a DSB within G1 cells is not sufficient to alter chromosome dynamics. However, DSBs formed within an asynchronous cell population result in large decreases in both intra- and interchromosomal interactions. Using live cell microscopy, we find that changes in chromosome dynamics correlate with relocalization of the DSB to the nuclear periphery. Sequestration to the periphery requires the nuclear envelope protein, Mps3p, and Mps3p-dependent tethering delays recombinational repair of a DSB and enhances gross chromosomal rearrangements. Furthermore, we show that components of the telomerase machinery are recruited to a DSB and that telomerase recruitment is required for its peripheral localization. Based on these findings, we propose that sequestration of unrepaired or slowly repaired DSBs to the nuclear periphery reflects a competition between alternative repair pathways.
Figures
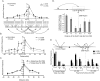
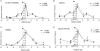
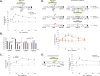
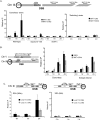
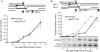
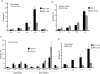
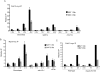
Similar articles
-
Asymmetric Processing of DNA Ends at a Double-Strand Break Leads to Unconstrained Dynamics and Ectopic Translocation.Cell Rep. 2018 Sep 4;24(10):2614-2628.e4. doi: 10.1016/j.celrep.2018.07.102. Cell Rep. 2018. PMID: 30184497
-
Telomere tethering at the nuclear periphery is essential for efficient DNA double strand break repair in subtelomeric region.J Cell Biol. 2006 Jan 16;172(2):189-99. doi: 10.1083/jcb.200505159. J Cell Biol. 2006. PMID: 16418532 Free PMC article.
-
Cell cycle-dependent spatial segregation of telomerase from sites of DNA damage.J Cell Biol. 2017 Aug 7;216(8):2355-2371. doi: 10.1083/jcb.201610071. Epub 2017 Jun 21. J Cell Biol. 2017. PMID: 28637749 Free PMC article.
-
Chromosome healing by de novo telomere addition in Saccharomyces cerevisiae.Mol Microbiol. 2006 Mar;59(5):1357-68. doi: 10.1111/j.1365-2958.2006.05026.x. Mol Microbiol. 2006. PMID: 16468981 Review.
-
Emerging non-canonical roles for the Rad51-Rad52 interaction in response to double-strand breaks in yeast.Curr Genet. 2020 Oct;66(5):917-926. doi: 10.1007/s00294-020-01081-z. Epub 2020 May 12. Curr Genet. 2020. PMID: 32399607 Free PMC article. Review.
Cited by
-
SUN1/2 controls macrophage polarization via modulating nuclear size and stiffness.Nat Commun. 2023 Oct 12;14(1):6416. doi: 10.1038/s41467-023-42187-5. Nat Commun. 2023. PMID: 37828059 Free PMC article.
-
The SUN-like protein TgSLP1 is essential for nuclear division in the apicomplexan parasite Toxoplasma gondii.J Cell Sci. 2023 Nov 1;136(21):jcs260337. doi: 10.1242/jcs.260337. Epub 2023 Oct 30. J Cell Sci. 2023. PMID: 37815466 Free PMC article.
-
Avidity-based biosensors for ubiquitylated PCNA reveal choreography of DNA damage bypass.Sci Adv. 2023 Sep 8;9(36):eadf3041. doi: 10.1126/sciadv.adf3041. Epub 2023 Sep 6. Sci Adv. 2023. PMID: 37672592 Free PMC article.
-
An Expansion of the Endoplasmic Reticulum that Halts Autophagy is Permissive to Genome Instability.Contact (Thousand Oaks). 2023 Feb 22;6:25152564231157706. doi: 10.1177/25152564231157706. eCollection 2023 Jan-Dec. Contact (Thousand Oaks). 2023. PMID: 37366415 Free PMC article.
-
Transmembrane nuclease NUMEN/ENDOD1 regulates DNA repair pathway choice at the nuclear periphery.Nat Cell Biol. 2023 Jul;25(7):1004-1016. doi: 10.1038/s41556-023-01165-1. Epub 2023 Jun 15. Nat Cell Biol. 2023. PMID: 37322289
References
-
- Antoniacci L.M., Kenna M.A., Uetz P., Fields S., Skibbens R.V. The spindle pole body assembly component Mps3p/Nep98p functions in sister chromatid cohesion. J. Biol. Chem. 2004;279:49542–49550. - PubMed
-
- Antoniacci L.M., Kenna M.A., Skibbens R.V. The nuclear envelope and spindle pole body-associated Mps3 protein bind telomere regulators and function in telomere clustering. Cell Cycle. 2007;6:75–79. - PubMed
-
- Aten J.A., Stap J., Krawczyk P.M., van Oven C.H., Hoebe R.A., Essers J., Kanaar R. Dynamics of DNA double-strand breaks revealed by clustering of damaged chromosome domains. Science. 2004;303:92–95. - PubMed
-
- Bakkenist C.J., Kastan M.B. DNA damage activates ATM through intermolecular autophosphorylation and dimer dissociation. Nature. 2003;421:499–506. - PubMed
Publication types
MeSH terms
Substances
Grants and funding
LinkOut - more resources
Full Text Sources
Other Literature Sources
Molecular Biology Databases