Glycolipid transfer protein interaction with bilayer vesicles: modulation by changing lipid composition
- PMID: 16169991
- PMCID: PMC1366967
- DOI: 10.1529/biophysj.105.070631
Glycolipid transfer protein interaction with bilayer vesicles: modulation by changing lipid composition
Abstract
Glycosphingolipids (GSLs) are important constituents of lipid rafts and caveolae, are essential for the normal development of cells, and are adhesion sites for various infectious agents. One strategy for modulating GSL composition in lipid rafts is to selectively transfer GSL to or from these putative membrane microdomains. Glycolipid transfer protein (GLTP) catalyzes selective intermembrane transfer of GSLs. To enable effective use of GLTP as a tool to modify the glycolipid content of membranes, it is imperative to understand how the membrane regulates GLTP action. In this study, GLTP partitioning to membranes was analyzed by monitoring the fluorescence resonance energy transfer from tryptophans and tyrosines of GLTP to N-(5-dimethyl-aminonaphthalene-1-sulfonyl)-1,2-dihexadecanoyl-sn-glycero-3-phospho-ethanolamine present in bilayer vesicles. GLTP partitioned to POPC vesicles even when no GSL was present. GLTP interaction with model membranes was nonpenetrating, as assessed by protein-induced changes in lipid monolayer surface pressure, and nonperturbing in that neither membrane fluidity nor order were affected, as monitored by anisotropy of 1,6-diphenyl-1,3,5-hexatriene and 6-dodecanoyl-N,N-dimethyl-2-naphthylamine, even though the tryptophan anisotropy of GLTP increased in the presence of vesicles. Ionic strength, vesicle packing, and vesicle lipid composition affected GLTP partitioning to the membrane and led to the following conclusion: Conditions that increase the ratio of bound/unbound GLTP do not guarantee increased transfer activity, but conditions that decrease the ratio of bound/unbound GLTP always diminish transfer. A model of GLTP interaction with the membrane, based on the partitioning equilibrium data and consistent with the kinetics of GSL transfer, is presented and solved mathematically.
Figures
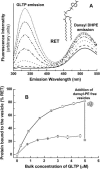
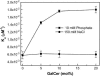
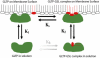
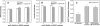
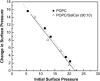
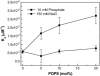
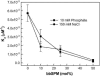
Similar articles
-
Glycolipid transfer proteins.Biochim Biophys Acta. 2007 Jun;1771(6):746-60. doi: 10.1016/j.bbalip.2007.01.011. Epub 2007 Jan 24. Biochim Biophys Acta. 2007. PMID: 17320476 Free PMC article. Review.
-
Glycolipid transfer protein mediated transfer of glycosphingolipids between membranes: a model for action based on kinetic and thermodynamic analyses.Biochemistry. 2004 Nov 2;43(43):13805-15. doi: 10.1021/bi0492197. Biochemistry. 2004. PMID: 15504043 Free PMC article.
-
Membrane interaction and activity of the glycolipid transfer protein.Biochim Biophys Acta. 2006 Nov;1758(11):1732-42. doi: 10.1016/j.bbamem.2006.06.020. Epub 2006 Jul 7. Biochim Biophys Acta. 2006. PMID: 16908009
-
Monitoring glycolipid transfer protein activity and membrane interaction with the surface plasmon resonance technique.Biochim Biophys Acta. 2011 Jan;1808(1):47-54. doi: 10.1016/j.bbamem.2010.08.018. Epub 2010 Sep 8. Biochim Biophys Acta. 2011. PMID: 20804726
-
Effects of membrane lipids on ion channel structure and function.Cell Biochem Biophys. 2003;38(2):161-90. doi: 10.1385/CBB:38:2:161. Cell Biochem Biophys. 2003. PMID: 12777713 Review.
Cited by
-
Sphingolipid transfer proteins defined by the GLTP-fold.Q Rev Biophys. 2015 Aug;48(3):281-322. doi: 10.1017/S003358351400016X. Epub 2015 Mar 23. Q Rev Biophys. 2015. PMID: 25797198 Free PMC article.
-
GLTP-fold interaction with planar phosphatidylcholine surfaces is synergistically stimulated by phosphatidic acid and phosphatidylethanolamine.J Lipid Res. 2013 Apr;54(4):1103-13. doi: 10.1194/jlr.M034744. Epub 2013 Jan 31. J Lipid Res. 2013. PMID: 23369752 Free PMC article.
-
Glycolipid transfer proteins.Biochim Biophys Acta. 2007 Jun;1771(6):746-60. doi: 10.1016/j.bbalip.2007.01.011. Epub 2007 Jan 24. Biochim Biophys Acta. 2007. PMID: 17320476 Free PMC article. Review.
-
Human GLTP: Three distinct functions for the three tryptophans in a novel peripheral amphitropic fold.Biophys J. 2010 Oct 20;99(8):2626-35. doi: 10.1016/j.bpj.2010.08.038. Biophys J. 2010. PMID: 20959104 Free PMC article.
-
ATP-binding cassette transporters mediate differential biosynthesis of glycosphingolipid species.J Lipid Res. 2021;62:100128. doi: 10.1016/j.jlr.2021.100128. Epub 2021 Sep 28. J Lipid Res. 2021. PMID: 34597626 Free PMC article.
References
-
- Bektas, M., and S. Spiegel. 2004. Glycosphingolipids and cell death. Glycoconj. J. 20:39–47. - PubMed
-
- Mañes, S., G. del Real, and C. Martínez-A. 2003. Pathogens: raft hijackers. Nature Rev. Immunol. 3:557–568. - PubMed
-
- Mahfoud, R., N. Garmy, M. Maresca, N. Yahi, A. Puigserver, and J. Fantini. 2002. Identification of a common sphingolipid-binding domain in Alzheimer, prion, and HIV-1 proteins. J. Biol. Chem. 277:11292–11294. - PubMed
-
- Simons, K., and E. Ikonen. 1997. Functional rafts in cell membranes. Nature. 387:569–572. - PubMed
Publication types
MeSH terms
Substances
Grants and funding
LinkOut - more resources
Full Text Sources