A crucial role of caspase-3 in osteogenic differentiation of bone marrow stromal stem cells
- PMID: 15599395
- PMCID: PMC535063
- DOI: 10.1172/JCI20427
A crucial role of caspase-3 in osteogenic differentiation of bone marrow stromal stem cells
Abstract
Caspase-3 is a critical enzyme for apoptosis and cell survival. Here we report delayed ossification and decreased bone mineral density in caspase-3-deficient (Casp3(-/-) and Casp3(+/-)) mice due to an attenuated osteogenic differentiation of bone marrow stromal stem cells (BMSSCs). The mechanism involved in the impaired differentiation of BMSSCs is due, at least partially, to the overactivated TGF-beta/Smad2 signaling pathway and the upregulated expressions of p53 and p21 along with the downregulated expressions of Cdk2 and Cdc2, and ultimately increased replicative senescence. In addition, the overactivated TGF-beta/Smad2 signaling may result in the compromised Runx2/Cbfa1 expression in preosteoblasts. Furthermore, we demonstrate that caspase-3 inhibitor, a potential agent for clinical treatment of human diseases, caused accelerated bone loss in ovariectomized mice, which is also associated with the overactivated TGF-beta/Smad2 signaling in BMSSCs. This study demonstrates that caspase-3 is crucial for the differentiation of BMSSCs by influencing TGF-beta/Smad2 pathway and cell cycle progression.
Figures
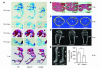
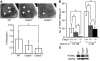
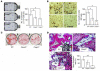
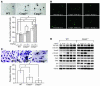
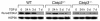
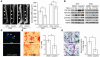
Similar articles
-
Extracellular matrix proteoglycans control the fate of bone marrow stromal cells.J Biol Chem. 2005 Aug 26;280(34):30481-9. doi: 10.1074/jbc.M500573200. Epub 2005 Jun 17. J Biol Chem. 2005. PMID: 15964849
-
Hypoxia inhibition of adipocytogenesis in human bone marrow stromal cells requires transforming growth factor-beta/Smad3 signaling.J Biol Chem. 2005 Jun 17;280(24):22688-96. doi: 10.1074/jbc.M412953200. Epub 2005 Apr 20. J Biol Chem. 2005. PMID: 15845540 Free PMC article.
-
Unique and redundant roles of Smad3 in TGF-beta-mediated regulation of long bone development in organ culture.Dev Dyn. 2004 Aug;230(4):685-99. doi: 10.1002/dvdy.20100. Dev Dyn. 2004. PMID: 15254903
-
hTERT transcription is repressed by Cbfa1 in human mesenchymal stem cell populations.J Bone Miner Res. 2007 Jun;22(6):897-906. doi: 10.1359/jbmr.070308. J Bone Miner Res. 2007. PMID: 17352650
-
Menin and TGF-beta superfamily member signaling via the Smad pathway in pituitary, parathyroid and osteoblast.Horm Metab Res. 2005 Jun;37(6):375-9. doi: 10.1055/s-2005-870152. Horm Metab Res. 2005. PMID: 16001330 Review.
Cited by
-
IFN-γ and TNF-α synergistically induce mesenchymal stem cell impairment and tumorigenesis via NFκB signaling.Stem Cells. 2013 Jul;31(7):1383-95. doi: 10.1002/stem.1388. Stem Cells. 2013. PMID: 23553791 Free PMC article.
-
Caspase-3 protects stressed organs against cell death.Mol Cell Biol. 2012 Nov;32(22):4523-33. doi: 10.1128/MCB.00774-12. Epub 2012 Sep 4. Mol Cell Biol. 2012. PMID: 22949508 Free PMC article.
-
Cocaine exposure in vitro induces apoptosis in fetal locus coeruleus neurons by altering the Bax/Bcl-2 ratio and through caspase-3 apoptotic signaling.Neuroscience. 2007 Jan 19;144(2):509-21. doi: 10.1016/j.neuroscience.2006.09.047. Epub 2006 Nov 3. Neuroscience. 2007. PMID: 17084983 Free PMC article.
-
Should we reconsider the apoptosis as a strategic player in tissue regeneration?Int J Biol Sci. 2019 Jul 25;15(10):2029-2036. doi: 10.7150/ijbs.36362. eCollection 2019. Int J Biol Sci. 2019. PMID: 31592227 Free PMC article. Review.
-
Physiological functions of caspases beyond cell death.Am J Pathol. 2006 Sep;169(3):729-37. doi: 10.2353/ajpath.2006.060105. Am J Pathol. 2006. PMID: 16936249 Free PMC article. Review. No abstract available.
References
-
- Strasser A, O’Connor L, Dixit VM. Apoptosis signaling. Annu. Rev. Biochem. 2000;69:217–245. - PubMed
-
- Nicholson DW. Caspase structure, proteolytic substrates, and function during apoptotic cell death. Cell Death Differ. 1999;6:1028–1042. - PubMed
-
- Kuida K, et al. Decreased apoptosis in the brain and premature lethality in CPP32-deficient mice. Nature. 1996;384:368–372. - PubMed
Publication types
MeSH terms
Substances
LinkOut - more resources
Full Text Sources
Other Literature Sources
Molecular Biology Databases
Research Materials
Miscellaneous