Targeted disruption of the mouse transforming growth factor-beta 1 gene results in multifocal inflammatory disease
- PMID: 1436033
- PMCID: PMC3889166
- DOI: 10.1038/359693a0
Targeted disruption of the mouse transforming growth factor-beta 1 gene results in multifocal inflammatory disease
Abstract
Transforming growth factor-beta 1 (TGF-beta 1) is a multifunctional growth factor that has profound regulatory effects on many developmental and physiological processes. Disruption of the TGF-beta 1 gene by homologous recombination in murine embryonic stem cells enables mice to be generated that carry the disrupted allele. Animals homozygous for the mutated TGF-beta 1 allele show no gross developmental abnormalities, but about 20 days after birth they succumb to a wasting syndrome accompanied by a multifocal, mixed inflammatory cell response and tissue necrosis, leading to organ failure and death. TGF-beta 1-deficient mice may be valuable models for human immune and inflammatory disorders, including autoimmune diseases, transplant rejection and graft versus host reactions.
Figures
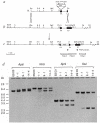
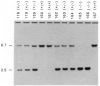
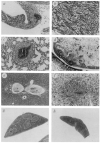
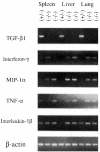
Similar articles
-
Onset and progression of pathological lesions in transforming growth factor-beta 1-deficient mice.Am J Pathol. 1995 Jan;146(1):276-88. Am J Pathol. 1995. PMID: 7856734 Free PMC article.
-
Early-onset multifocal inflammation in the transforming growth factor beta 1-null mouse is lymphocyte mediated.Proc Natl Acad Sci U S A. 1995 Dec 19;92(26):12215-9. doi: 10.1073/pnas.92.26.12215. Proc Natl Acad Sci U S A. 1995. PMID: 8618872 Free PMC article.
-
Transforming growth factor beta 1 (TGF-beta 1) controls expression of major histocompatibility genes in the postnatal mouse: aberrant histocompatibility antigen expression in the pathogenesis of the TGF-beta 1 null mouse phenotype.Proc Natl Acad Sci U S A. 1993 Nov 1;90(21):9944-8. doi: 10.1073/pnas.90.21.9944. Proc Natl Acad Sci U S A. 1993. PMID: 8234339 Free PMC article.
-
Transforming growth factor beta (TGF-beta) and autoimmunity.Autoimmun Rev. 2005 Sep;4(7):450-9. doi: 10.1016/j.autrev.2005.03.006. Autoimmun Rev. 2005. PMID: 16137611 Review.
-
Transforming growth factor-beta 1 in reproduction and development.Mol Reprod Dev. 1994 Oct;39(2):239-46. doi: 10.1002/mrd.1080390218. Mol Reprod Dev. 1994. PMID: 7826627 Review.
Cited by
-
Macrophage TGF-β signaling is critical for wound healing with heterotopic ossification after trauma.JCI Insight. 2022 Oct 24;7(20):e144925. doi: 10.1172/jci.insight.144925. JCI Insight. 2022. PMID: 36099022 Free PMC article.
-
Druggability of lipid metabolism modulation against renal fibrosis.Acta Pharmacol Sin. 2022 Mar;43(3):505-519. doi: 10.1038/s41401-021-00660-1. Epub 2021 May 14. Acta Pharmacol Sin. 2022. PMID: 33990764 Free PMC article. Review.
-
Reducing affinity of αvβ8 interactions with latent TGFβ: dialling down fibrosis.Ann Transl Med. 2015 May;3(Suppl 1):S31. doi: 10.3978/j.issn.2305-5839.2015.02.18. Ann Transl Med. 2015. PMID: 26046078 Free PMC article. No abstract available.
-
Salidroside alleviates paraquat-induced rat acute lung injury by repressing TGF-β1 expression.Int J Clin Exp Pathol. 2014 Dec 1;7(12):8841-7. eCollection 2014. Int J Clin Exp Pathol. 2014. PMID: 25674253 Free PMC article.
-
Enhancing Therapeutic Efficacy of FLT3 Inhibitors with Combination Therapy for Treatment of Acute Myeloid Leukemia.Int J Mol Sci. 2024 Aug 30;25(17):9448. doi: 10.3390/ijms25179448. Int J Mol Sci. 2024. PMID: 39273395 Free PMC article. Review.
References
-
- Barnard JA, Lyons RM, Moses HL. Biochlm biophys.Acta. 1990;1032:79–87. - PubMed
-
- Massague JA. Rev. Cell. Biol. 1990;6:597–641. - PubMed
-
- Roberts AB, Sporn MB. In: Peptide Growth Factors and Their Receptors I. Sporn MB, Roberts AB, editors. Springer; New York: 1990. pp. 419–472.
-
- Lehnert SA, Akhurst RJ. Development. 1988;104:263–273. - PubMed
Publication types
MeSH terms
Substances
Grants and funding
LinkOut - more resources
Full Text Sources
Other Literature Sources
Molecular Biology Databases