Receptor-mediated immunoglobulin G transport across mucosal barriers in adult life: functional expression of FcRn in the mammalian lung
- PMID: 12163559
- PMCID: PMC2193935
- DOI: 10.1084/jem.20020400
Receptor-mediated immunoglobulin G transport across mucosal barriers in adult life: functional expression of FcRn in the mammalian lung
Erratum in
- J Exp Med. 2003 Jun 2;197(11):1601
Abstract
Mucosal secretions of the human gastrointestinal, respiratory, and genital tracts contain the immunoglobulins (Ig)G and secretory IgA (sIgA) that function together in host defense. Exactly how IgG crosses epithelial barriers to function in mucosal immunity remains unknown. Here, we test the idea that the MHC class I-related Fc-receptor, FcRn, transports IgG across the mucosal surface of the human and mouse lung from lumen to serosa. We find that bronchial epithelial cells of the human, nonhuman primate, and mouse, express FcRn in adult-life, and demonstrate FcRn-dependent absorption of a bioactive Fc-fusion protein across the respiratory epithelium of the mouse in vivo. Thus, IgG, like dimeric IgA, can cross epithelial barriers by receptor-mediated transcytosis in adult animals. These data show that mucosal surfaces that express FcRn reabsorb IgG and explain a mechanism by which IgG may act in immune surveillance to retrieve lumenal antigens for processing in the lamina propria or systemically.
Figures
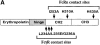
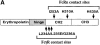
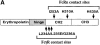
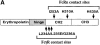
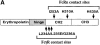
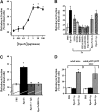
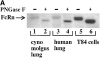
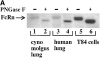
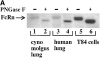
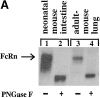
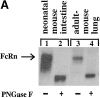
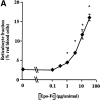
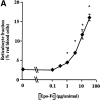
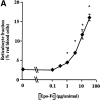
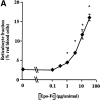
Similar articles
-
Bidirectional FcRn-dependent IgG transport in a polarized human intestinal epithelial cell line.J Clin Invest. 1999 Oct;104(7):903-11. doi: 10.1172/JCI6968. J Clin Invest. 1999. PMID: 10510331 Free PMC article.
-
Neonatal Fc Receptor Regulation of Lung Immunoglobulin and CD103+ Dendritic Cells Confers Transient Susceptibility to Tuberculosis.Infect Immun. 2016 Sep 19;84(10):2914-21. doi: 10.1128/IAI.00533-16. Print 2016 Oct. Infect Immun. 2016. PMID: 27481246 Free PMC article.
-
IgG transport across mucosal barriers by neonatal Fc receptor for IgG and mucosal immunity.Springer Semin Immunopathol. 2006 Dec;28(4):397-403. doi: 10.1007/s00281-006-0054-z. Epub 2006 Oct 19. Springer Semin Immunopathol. 2006. PMID: 17051393
-
The neonatal Fc receptor in mucosal immune regulation.Scand J Immunol. 2021 Feb;93(2):e13017. doi: 10.1111/sji.13017. Epub 2021 Jan 17. Scand J Immunol. 2021. PMID: 33351196 Review.
-
New functions of the MHC class I-related Fc receptor, FcRn.Biochem Soc Trans. 1997 May;25(2):481-6. doi: 10.1042/bst0250481. Biochem Soc Trans. 1997. PMID: 9191140 Review.
Cited by
-
Impact of mAb-FcRn affinity on IgG transcytosis across human well-differentiated airway epithelium.Front Immunol. 2024 Sep 16;15:1371156. doi: 10.3389/fimmu.2024.1371156. eCollection 2024. Front Immunol. 2024. PMID: 39351230 Free PMC article.
-
Analysis of Beyfortus® (Nirsevimab) Immunization Campaign: Effectiveness, Biases, and ADE Risks in RSV Prevention.Curr Issues Mol Biol. 2024 Sep 18;46(9):10369-10395. doi: 10.3390/cimb46090617. Curr Issues Mol Biol. 2024. PMID: 39329969 Free PMC article. Review.
-
Evaluation of a novel intramuscular prime/intranasal boost vaccination strategy against influenza in the pig model.PLoS Pathog. 2024 Aug 8;20(8):e1012393. doi: 10.1371/journal.ppat.1012393. eCollection 2024 Aug. PLoS Pathog. 2024. PMID: 39116029 Free PMC article.
-
SARS-CoV-2 Vaccines: The Advantage of Mucosal Vaccine Delivery and Local Immunity.Vaccines (Basel). 2024 Jul 18;12(7):795. doi: 10.3390/vaccines12070795. Vaccines (Basel). 2024. PMID: 39066432 Free PMC article. Review.
-
COVID-19 mRNA vaccines induce robust levels of IgG but limited amounts of IgA within the oronasopharynx of young children.medRxiv [Preprint]. 2024 Apr 16:2024.04.15.24305767. doi: 10.1101/2024.04.15.24305767. medRxiv. 2024. Update in: J Infect Dis. 2024 Sep 10:jiae450. doi: 10.1093/infdis/jiae450. PMID: 38699375 Free PMC article. Updated. Preprint.
References
-
- Holmgren, J., and A. Rudin. 1999. Mucosal immunity and bacteria. Mucosal Immunology. P.L. Ogra, J. Mestecky, M.E. Lamm, W. Strober, J. Bienenstock and J.R. McGhee, editors. Academic Press, San Diego, CA. pp. 685–693.
-
- Johansen, F.E., M. Pekna, I.N. Norderhaug, B. Haneberg, M.A. Hietala, P. Krajci, C. Betsholtz, and P. Brandtzaeg. 1999. Absence of epithelial immunoglobulin A transport, with increased mucosal leakiness, in polymeric immunoglobulin receptor/secretory component-deficient mice. J. Exp. Med. 190:915–922. - PMC - PubMed
-
- Robert-Guroff, M. 2000. IgG surfaces as an important component in mucosal protection. Nat. Med. 6:129–130. - PubMed
-
- Baba, T.W., V. Liska, R. Hofmann-Lehmann, J. Vlasak, W. Xu, S. Ayehunie, L.A. Cavacini, M.R. Posner, H. Katinger, G. Stiegler, et al. 2000. Human neutralizing monoclonal antibodies of the IgG1 subtype protect against mucosal simian-human immunodeficiency virus infection. Nat. Med. 6:200–206. - PubMed
Publication types
MeSH terms
Substances
Grants and funding
LinkOut - more resources
Full Text Sources
Other Literature Sources
Research Materials
Miscellaneous