Molecular proximity of Kv1.3 voltage-gated potassium channels and beta(1)-integrins on the plasma membrane of melanoma cells: effects of cell adherence and channel blockers
- PMID: 12084773
- PMCID: PMC2311400
- DOI: 10.1085/jgp.20028607
Molecular proximity of Kv1.3 voltage-gated potassium channels and beta(1)-integrins on the plasma membrane of melanoma cells: effects of cell adherence and channel blockers
Abstract
Tumor cell membranes have multiple components that participate in the process of metastasis. The present study investigates the physical association of beta1-integrins and Kv1.3 voltage-gated potassium channels in melanoma cell membranes using resonance energy transfer (RET) techniques. RET between donor-labeled anti-beta1-integrin and acceptor-labeled anti-Kv1.3 channels was detected on LOX cells adherent to glass and fibronectin-coated coverslips. However, RET was not observed on LOX cells in suspension, indicating that molecular proximity of these membrane molecules is adherence-related. Several K(+) channel blockers, including tetraethylammonium, 4-aminopyridine, and verapamil, inhibited RET between beta1-integrins and Kv1.3 channels. However, the irrelevant K(+) channel blocker apamin had no effect on RET between beta1-integrins and Kv1.3 channels. Based on these findings, we speculate that the lateral association of Kv1.3 channels with beta1-integrins contributes to the regulation of integrin function and that channel blockers might affect tumor cell behavior by influencing the assembly of supramolecular structures containing integrins.
Figures
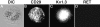
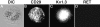
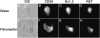
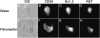
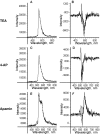
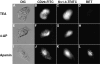
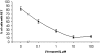
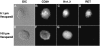
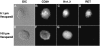
Similar articles
-
Extracellular K(+) and opening of voltage-gated potassium channels activate T cell integrin function: physical and functional association between Kv1.3 channels and beta1 integrins.J Exp Med. 2000 Apr 3;191(7):1167-76. doi: 10.1084/jem.191.7.1167. J Exp Med. 2000. PMID: 10748234 Free PMC article.
-
Predominant expression of Kv1.3 voltage-gated K+ channel subunit in rat prostate cancer cell lines: electrophysiological, pharmacological and molecular characterisation.Pflugers Arch. 2003 Aug;446(5):559-71. doi: 10.1007/s00424-003-1077-0. Epub 2003 Jul 1. Pflugers Arch. 2003. PMID: 12838421
-
Voltage-gated K+ channels in rat small cerebral arteries: molecular identity of the functional channels.J Physiol. 2003 Sep 15;551(Pt 3):751-63. doi: 10.1113/jphysiol.2003.040014. Epub 2003 Jun 18. J Physiol. 2003. PMID: 12815189 Free PMC article.
-
Potassium channels as therapeutic targets for autoimmune disorders.Curr Opin Drug Discov Devel. 2003 Sep;6(5):640-7. Curr Opin Drug Discov Devel. 2003. PMID: 14579513 Review.
-
Potassium channel-blockers as therapeutic agents to interfere with bone resorption of periodontal disease.J Dent Res. 2005 Jun;84(6):488-99. doi: 10.1177/154405910508400603. J Dent Res. 2005. PMID: 15914584 Review.
Cited by
-
Cells move when ions and water flow.Pflugers Arch. 2007 Jan;453(4):421-32. doi: 10.1007/s00424-006-0138-6. Epub 2006 Oct 5. Pflugers Arch. 2007. PMID: 17021798 Review.
-
K+ Channel Inhibition Differentially Regulates Migration of Intestinal Epithelial Cells in Inflamed vs. Non-Inflamed Conditions in a PI3K/Akt-Mediated Manner.PLoS One. 2016 Jan 29;11(1):e0147736. doi: 10.1371/journal.pone.0147736. eCollection 2016. PLoS One. 2016. PMID: 26824610 Free PMC article.
-
A stepwise neuron model fitting procedure designed for recordings with high spatial resolution: Application to layer 5 pyramidal cells.J Neurosci Methods. 2018 Jan 1;293:264-283. doi: 10.1016/j.jneumeth.2017.10.007. Epub 2017 Oct 7. J Neurosci Methods. 2018. PMID: 28993204 Free PMC article.
-
Aquaporins and Ion Channels as Dual Targets in the Design of Novel Glioblastoma Therapeutics to Limit Invasiveness.Cancers (Basel). 2023 Jan 30;15(3):849. doi: 10.3390/cancers15030849. Cancers (Basel). 2023. PMID: 36765806 Free PMC article. Review.
-
Tumor-reducing effect of the clinically used drug clofazimine in a SCID mouse model of pancreatic ductal adenocarcinoma.Oncotarget. 2017 Jun 13;8(24):38276-38293. doi: 10.18632/oncotarget.11299. Oncotarget. 2017. PMID: 27542263 Free PMC article.
References
-
- Albelda, S.M. 1993. Biology of disease. Role of integrins and other cell adhesion molecules in tumor progression and metastasis. Lab. Invest. 68:4–17. - PubMed
-
- Arcangeli, A., M.R. Del Bene, R. Poli, L. Ricupero, and M. Olivotto. 1989. Mutual contact of murine erythroleukemia cells activates depolarizing cation channels, whereas contact with extracellular substrata activates hyperpolarizing Ca2+-dependent K+ channels. J. Cell. Physiol. 139:1–8. - PubMed
-
- Arcangeli, A., A. Becchetti, M.R. Bene, E. Wanke, and M. Olivotto. 1991. Fibronectin-integrin binding promotes hyperpolarization of murine erythroleukemia cells. Biochem. Biophys. Res. Comm. 177:1266–1272. - PubMed
-
- Arcangeli, A., L. Faravelli, L. Bianchi, B. Rosati, A. Gritti, A. Vescovi, E. Wanke, and M. Olivotto. 1996. Soluble or bound laminin elicit in human neuroblastoma cells short- or long-term potentiation of a K+ inwardly rectifying current: relevance to neuritogenesis. Cell Adhes. Commun. 4:369–385. - PubMed
Publication types
MeSH terms
Substances
Grants and funding
LinkOut - more resources
Full Text Sources
Medical