Attenuation of the self-renewal of transit-amplifying osteoblast progenitors in the murine bone marrow by 17 beta-estradiol
- PMID: 11285299
- PMCID: PMC199573
- DOI: 10.1172/JCI11653
Attenuation of the self-renewal of transit-amplifying osteoblast progenitors in the murine bone marrow by 17 beta-estradiol
Abstract
In agreement with evidence that estrogens slow the rate of bone remodeling by suppressing the production of both osteoclasts and osteoblasts, loss of estrogens leads to an increase in the number of osteoclast as well as early osteoblast progenitors (CFU-osteoblasts; CFU-OBs) in the murine bone marrow. Here we show that CFU-OBs are early transit-amplifying progenitors, i.e., dividing cells capable of limited self-renewal, and that 17 beta-estradiol acts in vivo and in vitro to attenuate their self-renewal by approximately 50%. Consistent with a direct receptor-mediated action of estrogens on early mesenchymal cell progenitors, anti-estrogen receptor-alpha (anti-ER alpha) Ab's stain a small number of marrow cells that exhibit characteristics of primitive undifferentiated cells, including a high nucleus/cytoplasm ratio and lack of lineage-specific biochemical markers; the effect of 17 beta-estradiol on CFU-OB self-renewal is absent in mice lacking ER alpha. Because both osteoblasts and the stromal/osteoblastic cells that are required for osteoclast development are derived from CFU-OBs, suppression of the self-renewal of this common progenitor may represent a key mechanism of the anti-remodeling effects of estrogens.
Figures
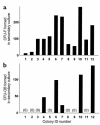
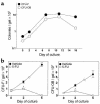
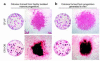
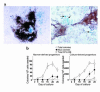
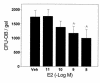
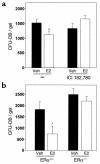
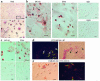
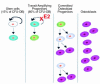
Similar articles
-
The estrogen receptor-alpha in osteoclasts mediates the protective effects of estrogens on cancellous but not cortical bone.Mol Endocrinol. 2010 Feb;24(2):323-34. doi: 10.1210/me.2009-0354. Epub 2010 Jan 6. Mol Endocrinol. 2010. PMID: 20053716 Free PMC article.
-
Estrogen promotes early osteoblast differentiation and inhibits adipocyte differentiation in mouse bone marrow stromal cell lines that express estrogen receptor (ER) alpha or beta.Endocrinology. 2002 Jun;143(6):2349-56. doi: 10.1210/endo.143.6.8854. Endocrinology. 2002. PMID: 12021200
-
Estradiol and raloxifene decrease the formation of multinucleate cells in human bone marrow cultures.Eur Cytokine Netw. 2002 Jan-Mar;13(1):39-45. Eur Cytokine Netw. 2002. PMID: 11956019
-
Cellular and molecular effects of growth hormone and estrogen on human bone cells.APMIS Suppl. 1997;71:1-30. APMIS Suppl. 1997. PMID: 9357492 Review.
-
New insights into the cellular, biochemical, and molecular basis of postmenopausal and senile osteoporosis: roles of IL-6 and gp130.Int J Immunopharmacol. 1995 Feb;17(2):109-16. doi: 10.1016/0192-0561(94)00089-7. Int J Immunopharmacol. 1995. PMID: 7657404 Review.
Cited by
-
FoxO-mediated defense against oxidative stress in osteoblasts is indispensable for skeletal homeostasis in mice.Cell Metab. 2010 Feb 3;11(2):136-46. doi: 10.1016/j.cmet.2009.12.009. Cell Metab. 2010. PMID: 20142101 Free PMC article.
-
Non-nuclear-initiated actions of the estrogen receptor protect cortical bone mass.Mol Endocrinol. 2013 Apr;27(4):649-56. doi: 10.1210/me.2012-1368. Epub 2013 Feb 26. Mol Endocrinol. 2013. PMID: 23443267 Free PMC article.
-
Physiological and pathophysiological bone turnover - role of the immune system.Nat Rev Endocrinol. 2016 Sep;12(9):518-32. doi: 10.1038/nrendo.2016.91. Epub 2016 Jun 17. Nat Rev Endocrinol. 2016. PMID: 27312863 Free PMC article. Review.
-
The YAP/TAZ transcriptional co-activators have opposing effects at different stages of osteoblast differentiation.Bone. 2018 Jul;112:1-9. doi: 10.1016/j.bone.2018.04.001. Epub 2018 Apr 4. Bone. 2018. PMID: 29626544 Free PMC article.
-
Mice with sclerostin gene deletion are resistant to the severe sublesional bone loss induced by spinal cord injury.Osteoporos Int. 2016 Dec;27(12):3627-3636. doi: 10.1007/s00198-016-3700-x. Epub 2016 Jul 20. Osteoporos Int. 2016. PMID: 27436301
References
-
- Cosman, F., Dempster, D., and Lindsay, R. 1997. Clinical effects of estrogens and antiestrogens on the skeleton and skeletal metabolism. In Estrogens and antiestrogens. R. Lindsay, D.W. Dempster, and V.C. Jordan, editors. Lippincott-Raven Publishers. Philadelphia, Pennsylvania, USA. 151–164.
-
- Parfitt AM. Osteonal and hemi-osteonal remodeling: the spatial and temporal framework for signal traffic in adult human bone. J Cell Biochem. 1994;55:273–286. - PubMed
-
- Eriksen EF, et al. Cancellous bone remodeling in type I (postmenopausal) osteoporosis: quantitative assessment of rates of formation, resorption, and bone loss at tissue and cellular levels. J Bone Miner Res. 1990;5:311–319. - PubMed
Publication types
MeSH terms
Substances
Grants and funding
LinkOut - more resources
Full Text Sources
Other Literature Sources
Medical
Miscellaneous